A response to economists who doubt our capacity to decarbonize while maintaining robust growth
In this piece, Michael Grubb responds to INET research by Gregor Semieniuk, Lance Taylor, and Armon Rezai and Enno Schröder and Servaas Storm, arguing that “conditional optimism” rather than pessimism is how economists should view the prospect of growth under decarbonization.
1. Introduction
The Paris Agreement on climate change, the escalating evidence of direct, observable and damaging climate change impacts, and the IPCC’s recent report on “1.5 degrees” have stimulated a new surge of economic analysis. When INET invited me to write a response of two new papers on the topic, I jumped at the chance because both are by top-level economists, notable for injecting rigorous new analysis and evidence into sometimes sterile debates.
Both papers express a profound concern about the seriousness of climate change, and indeed reveal barely-concealed anguish at the path they find us to be on. For their analysis leads both teams to the – only slightly caveated - conclusion that the emission reductions required to the deliver the Paris Aims (“well below 2 deg.C”) are implausible, by almost any standard of macroeconomic evidence – and still more so for the most ambitious “1.5 deg.C” end of the spectrum.
The paper by Schröder and Storm – “.. paved with good intentions” – breaks the problem down into components and examines the maximum rates of changed historically observed in each, finding that the IPCC scenarios represent wholly unprecedented rates of change. The paper by Semieniuk, Taylor and Rezai finds that the energy demand reductions central notably to the IPCC 1.5C scenarios - are implausibly and “overly optimistic about global productivity growth”, based on a more explicit macro model. To try and dilute the pessimism they seek refuge in the hope of a huge supply-side transition, costing several percent of GDP, of precisely the kind that Schroeder and Storm conclude is implausible based on historical experience.
With this paper I want to give my economist colleagues a bit more hope. I do so in a rather unusual way – by setting out why, despite agreeing with many aspects of their analysis, I think they err at the most fundamental level – in the underlying structural approach. With the wrong mental model, they bring to the table the wrong form of maths and thereby to an apparently mathematically objective pessimism. The Paris targets are unquestionable challenging, but I argue that a more robust approach leads to a more nuanced, conditional and interesting outlook.
More broadly, I offer three distinct suggestions for wider consideration. The first, the main focus, is a different mental and mathematical framework through which to interpret observed trends. Second, I argue that to derive policy implications from the historical record requires understanding the processes of energy and economic development in terms of three components – domestic infrastructure, internationally mobile manufacturing, and consumption – the relative significance of which varies with stages of economic development. Third, I suggest that the papers, and this response, may be emblematic of a growing and necessary trend in energy-climate economics, of what we might term historical futures analysis. Whilst this has potential to be a clear improvement on previous approaches, I suggest four principles that should inform such studies – and offer one tentative conclusion.
Together these form the bedrock of my “conditional optimism”: conditional, in the sense that the recent Nobel Laureate Paul Romer so neatly encapsulated with his fable of a child wanting to build a treehouse.[1]
Complacent optimism is the spoilt child who sits and complains if his parents do not build (or have built) the treehouse he wants. Conditional optimism is the child who says: get me the wood, the hammer, the nails and the guidebook, and I can build a treehouse.
2. The Basis for Pessimism
As noted, both papers are characterized by concern about climate change and the urgency of response: they express not skepticism about the problem, but pessimism about our ability to change what matters fast enough. Both use historical data as their bedrock, and focus on rates of change. I regard this as a positive step, far better than the fantasies of earlier modeling which made exogenous projections of future ‘autonomous energy efficiency gains’, and technology costs over the rest of the Century, with scant evidence; and then applied them to theoretical models which assumed some globally rational, all-powerful decision making with (usually perfect) foresight. Many such models contained no inertia or dynamics, being based on an implausible series of sequential ‘equilibrium’ conditions.
The exchange here will be seen to have some relevance to such models, but in general I regard the move to empirically grounded approaches that seek evidence concerning rates of change as an intellectual step forward. However as the saying goes, a little knowledge can be a dangerous thing.
Schröder and Storm are simultaneously thoughtful, clear and frustrating. Their analysis is thoughtful in warning about specific theoretical models and is rigorously clear in consequently breaking down into the four components of the Kaya identity. From this they conclude that the IPCC scenarios require unprecedented percentage rates of change, in energy or carbon intensity, if economic growth is not to be constrained; and find no evidence in the historical record that this is possible.
Semieniuk et al adopt a more explicit macro-economic perspective to focus on energy intensity trends and constraints, offering powerful arguments which if anything reinforce Schröder and Storm’s scepticism about accelerating energy efficiency as the way out - particularly to the degree posited in the IPCC 1.5C scenarios, which envision a major reduction in global energy demand over the next few decades. For both papers, the fundamental indicator of the main components is the % rate of change observed historically.
A key to econometrically-grounded analysis is to understand the structure and main drivers. Thus:
the rate of decarbonization in the OECD has been slowing down during 1991-2015 compared to the years 1971-1990.
Of course: the earlier period reflects response to the massive oil shocks of the 1970s, whereas the period since 1990 was a period of low energy prices and the decline of nuclear power, previously the main driver of decarbonization.
… The E.U. carbon intensity decline recorded during 1991-2015 is dominated by the growing share of (zero-carbon) renewables in total energy use..
Which is the core focus I turn to. So let me first radically simplify my response to the Schröder and Storm framework by assuming that two of the four factors in their Kaya identity breakdown – population and GDP growth – are predetermined as projected. To really zero in on the core issue I will also start by taking global energy demand as given (I turn to energy efficiency more briefly later), so as to focus my main point squarely on the issue of the carbon intensity of energy supply.
The metric the papers focuses on is how fast this can change. This is indeed highly relevant, but it is simply a result of what I argue is much more fundamental: the rate of displacement of carbon intensive supply by the growth of alternate sources. The analytic driver should be not on the rate of carbon intensity reduction, but on the rates and processes of substitution by cleaner energy sources.
3. The Analytics of Conditional Optimism: Logistic Substitution
This has rather interesting and profound consequences and I need to clarify what I mean as substitution. Economics tends to use the term in relation to substitution between different factors of production. Here I am talking about technology substitutions for essentially the same factor of production – energy.
Substitution between factors of production is typically represented by elasticities, a broadly static (and reversible) concept based on how relative price changes affect the use of different factors. Technology substitution however in reality is an intrinsically dynamic and largely irreversible concept, which focuses on the endogenous cost reductions and market growth of new technological systems as they displace older, incumbent industries.
In the language of our book Planetary Economics, the former is an essentially second domain concept, based on exogenously defined technology parameters, striving towards a least-cost equilibrium; the latter is an intrinsically dynamic and evolutionary concept in which technologies are “striving” for dominance.
3.1 The Structure of Logistic Technology Penetration
The processes mapped out in the associated literature have a well-established mathematical form of logistic substitution - otherwise known as penetration or S-curves - of which Figure 1(a) shows the simplest possible case of direct and total substitution of one product by another. The functional form is usually described as:

Where MAX is the maximum penetration and k determines the rate and MAX/(1+a) determines the amount deployed at time t relative to the date t0 (negative t being before this “anchor date”). The larger is a, the longer the new technology sustains an exponential growth before this slows. The higher is k, the faster the main transition. MAX=1 represents the case of total substitution shown for simplicity in Figure 1.
The dynamic parameters I used for Figure 1(a) are ones that seem to reflect simultaneously the observed timescales of past major energy transitions (such as the 2-3 decade timescales of structural adjustments to the oil price shocks: Bashmakov et al, 2018); and the observed growth rates of the main new renewable energy sources over the past fifteen years (at 20-30% annually, declining for wind energy as installed capacities reach 10-20% of installed capacity). (Specifically: a=2.5 and k = 0.25, with MAX=1). In terms of these renewable sources, for simplicity one can consider the zero point in Figure 1 as being about 2015, so that -15 goes back to the year 2000. As with the INET papers discussed here, the parameter choices can thus be equally concerned with what we know about historical energy trends, but they lead to a radically different view of future possibilities.
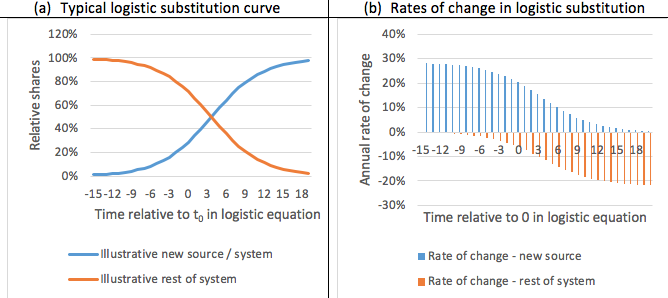
Figure 1: Classical Logistic Penetration Curve Note: Generated with classic logistics curve (equation 1), parameter values: a=2.5 and k = 0.25, with MAX=1
At this stage the figure is just an illustration to give a sense of dynamics. Of course the percentage growth rate of the new entrant declines, but only once its share has become significant, and then dominant. In this most simple illustrative case, note that at time t=-1 – which consider our most recent observable data at time t - the new entrant has reached just over 20% of the market with a growing impact on the incumbent, which has reached a till-then unprecedented decline of 5%/yr. But ‘From little acorns do mighty oaks grow’, and by the end of the 35 year time period, the incumbent industry is driven out by the newcomer, declining at rates exceeding 20%/yr in the latter stages.
This illustration is simply intended to illustrate the dynamic form, given the parameter values, but nevertheless contrasts rather dramatically with the average rate of decarbonization “ = ─3.68%/yr” observed in the IPCC scenarios and described in Schoeder and Storm as “very aggressive de-carbonization” and taken as the “very optimistic” constraint that drives their conclusions. In the simplistic transition of Figure 1, it takes 12 years to reach this rate of decline, but the transition gathers pace. Averaged over the full 35 years, a constant percentage reduction would require c = - 10%/yr to reach the same end-point – almost impossible at the starting point, but entirely feasible and easily observed in the latter stages of sunset industries.
In short, the conclusions we draw from historical data depend entirely upon what kind of process we think we are trying to measure: rates of historical decline, or rates of new growth; and equally, economic factor substitution driven by externality costs with relatively constant technologies, or a dynamic substitution process driven by innovation and scale economies displacing incumbent industries.
Another crucial and, to many economists, even more disconcerting point flows from this: more data, incorrectly interpreted, is not always better. Both the INET papers draw on data going back to about 1970 (and Semieniuk et al discuss earlier trends). For the record, I agree: decarbonization in this data is almost unobservable and globally almost ceased from the 1990s after the oil scare waned and nuclear power slowed. But if one makes simple extrapolations of aggregate trends, concerning what is actually a system embarking on a logistics-type transition, the further back one goes – the longer the time series used to calibrate the assumed macro-relationships - the more misleading will be the aggregate numbers obtained.
3.2 Key Examples
Global PV. The world of course does not comprise a single source of CO2 being logistically displaced by a single zero carbon alternative. For its potential future global impact, probably nothing can surpass the solar revolution – for revolution it is, which has defied all predictions (even by Greenpeace). Ever since Germany launched its Energiewende in 2001, solar PV has seen sustained market growth at 30-40%/yr, and costs have fallen by a factor of 10 in less than a decade. The latest contracted prices show that PV and/or wind are the cheapest electricity sources in rapidly increasingly swathes of the world (Lazard, 2018).[2]
PV is currently a trivial component of global electricity supplies, but a childishly simple extrapolation would hold that if PV could sustain growth at 30%/year, it would displace all carbon-based electricity by about 2040.[3]
It won’t, of course, because many factors (its diurnal and seasonal intermittency probably being more important than land, latitude or simple energy costs) will constrain penetration; and as indicated by the logistics curve, growth will slow considerably as the scale extents past half this level. But even a more considered logistics penetration process could make a substantial dent in global energy almost as quickly.
The UK electricity transition. In some ways, a more interesting, developed and telling example is the UK electricity transition. As befits an “island of coal in a sea of oil and gas”, the UK power in the early
1990s was about 80% coal generation. Twenty-five years later, coal is less than 10% of generation and the UK’s power sector CO2 emissions have halved.[4]
Figure 2 shows a stylized representation of the transition so far, and a projection based on logistic curve parameters as illustrated in Figure 1. For simplicity, with t=0 in the charts taken as the year 2000, then the figure shows three technologies parametrized as:
- Gas penetration into the system, displacing coal, with a logistic anchor t0 = 2005 and a maximum theoretical penetration at 75% of the system;
- Renewables penetrate into the system with a logistics anchor displaced 10 years to t0 = 2015, with a maximum penetration at 50% of the system;[5]
- “System” technologies penetrate into the system displaced another ten years (anchor t0=2025); this could comprise a suite of technologies ranging from ‘baseload zero carbon’ (nuclear, biomass or CCS) to storage and load management that enables the system to absorb more renewables over time, plus interconnectors that draw on foreign renewables and/or nuclear.
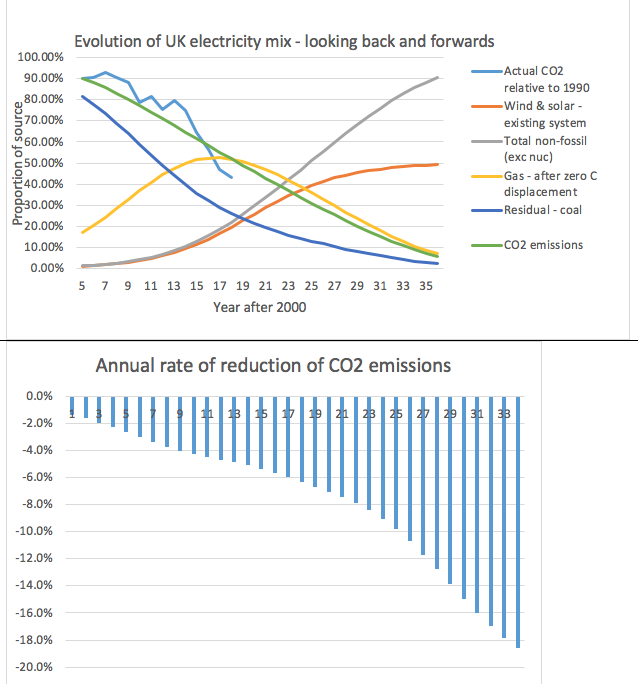
Figure 2 Evolution of UK electricity with and logistic extrapolation to 2035
Gas has about half the carbon intensity of coal, and for simplicity I assume that renewables+clean system technologies displace coal and gas in proportion to their respective generation at a given point in time; this gives a reasonable stylised approximation of UK electricity trends since 2000, including the fact that CO2 emission have dropped about 45% since 2000, with recent CO2 reductions at about 5%/yr.
But the extrapolation of this simple 4-technology model is that emissions could continue to decline at approximately the same linear rate – implying a steadily accelerating percentage rate - until by 2035 UK CO2 emissions are a tenth of the 2000 levels. As mapped out in far more detailed energy systems analysis by the UK’s climate change committee) electricity would then be growing rapidly into the transport sector – again, with the dynamics of logistic substitution – with vehicle batteries in turn becoming part of the storage and balancing capacity of the electricity system.
The difference from a perspective which just looks at the maximum CO2 reduction rate in the past, and sets that as an eternal constraint, could hardly be more stark.
.. and transport. The evidence for such transition extending into the transport sector is indeed already with us. In California – which has recently mandated a 100% renewables electricity sector by 2045 – the sales of electric vehicles have grown, from a trivial level of about 50,000 vehicles in 2012, at about 30% year, before a jump in 2018 (estimated on monthly sales data) to about 350,000 vehicles.[6] Given air quality considerations, a major policy debate internationally now is when city, state or national governments ban the sale of petroleum-based vehicles – already set in the 2030s in several European countries, but with Beijing for example debating dates in the mid 2020s.
3.3 A Markets Perspective
Moreover, a markets-based perspective only reinforces the message. In economic terms the decline of an industry should be measured in terms of its profitability, not its volume. And profitability generally declines as soon as the market stops growing (and vice-versa, of course). That can easily occur at only 5-10% penetration of competition. The European utilities that tried to ignore the energy transition are now economic zombies; some split their companies in two to try and isolate the assets that have already turned into liabilities in a decarbonizing system.
Under the onslaught of new energy sources and environmental pressures, the economics of coal generation is collapsing around the world. Trump’s best efforts have been unable to shore up the US coal industry, and even in Asia the statistics on new coal generation are becoming more notable for the number of planned projects being cancelled, than for those actually built – though of course risks remain, most obviously in Indian plans and projections for coal expansion. The inertia of incumbency—and the desperation of coal plan manufacturers to find new markets—is remarkable, but it ultimately cannot stop the forces of Schumpeterian “creative destruction,” now that clearly better alternatives have been developed and demonstrated.
And in that process, the “conditionally optimistic” model for projections should be one based on logistic growth of new sources and the associated “creative destruction” starting to bear down on the old. The future indeed needs to be different from the past; and past data does not say it cannot be so, indeed it already holds the evidence to suggest that it will be.
3.4 Conclusions on the Economics of Transition
So it really is about the economics of innovation-and-policy driven technological substitution. The paper by Schröder and Storm, seems almost schizophrenic in this—but recognizes the essential issue:
Without a concerted (global) policy shift to deep de-carbonization (Fankhauser and Jotzo 2017; Geels et al. 2017), a rapid transition to renewable energy sources (Peters et al. 2017), structural change in production, consumption and transportation (Steffen et al. 2018), and a transformation of finance (Malm 2016; Mazzucato and Semieniuk 2018), the decoupling will not even come close to what is needed (e.g. Storm 2017). The key insight is that marginal, incremental, improvements in energy and carbon efficiency cannot do the job and that what is needed is a structural transformation—and establishment economics lacks the instruments and approaches to analyze exactly this (Storm 2015; Wade 2018).
This is a great paragraph, and I agree with all-but-one word. But before I come to that word, there are two absolutely central points to make about it. The first half of the paragraph pinpoints four major challenges of which each seems like a vast task, and the combination hardly plausible. My suggestion is that in fact that they are all part of the same task, and that when you consider all four elements you conclude that this makes it easier, not harder: because all four are interlocking and mutually reinforcing.
Consider the renewables revolution—and by any standard it is a revolution even if people haven’t realized it yet. It involved (i) a major policy shift—serious use of feed-in tariffs in Germany. That has now led directly to (ii) a rapid transition towards renewable energy sources: by most standards, sustained growth of a new product at 20-30% or more a year is pretty rapid. This is now leading to a structural change in (iii-a) production of electricity—the entire system is changing to accommodate this dispersed, localized and intermittent source at scale, (iii-b) consumption, as households with PV on the roof have been shown to take a greater interest in their consumption of what was previously an entirely bland product; and iii-c) transportation, as electric vehicles will get a new boost from the enhanced value of storage services back to the grid. Finally, it involved the German Landesbank funding a huge scale of investment with low cost loans because they trusted German industry and the German political and legal system that lay behind the Energiewende, which of course then made wind and PV, as capital-intensive technologies, cheaper. And, note, once the founding contracts expire all this capital will continue to produce energy far more cheaply than any fossil fuel source.
So second, “The key insight is that marginal, incremental improvements in energy and carbon efficiency cannot do the job and that what is needed is a structural transformation”—agreed. And “establishment economics lacks the instruments and approaches to analyze exactly this (Storm 2015; Wade 2018)” just begs the question—if true, then why “establishment” economics—which must mean broadly second domain economics in the terminology of Planetary Economics—ignores what is perfectly well known and attested in the fields of technology studies and evolutionary and institutional economics. It is surely this “third domain” of economics which is most relevant to the questions at hand, and which—contrary to common assertions—can lend itself to empirically grounded, quantitative techniques which show just how much the future can be different from the past.
One response, of course, is that the analysis above is grounded in examples and sector/technology—not the whole thing. But that is the whole point. The literature on evolutionary economics underlines precisely that the world of the future grows from the niche markets of the present; and that in fields like energy, those options developments and niche markets which enable new sources to grow and challenge the huge power of incumbents have to be initially driven by public policies—as they have been. Finally, the role of markets—both economic markets and the social market of ideas and policies—is then not to settle in some non-existent equilibrium, but is to select, foster, and fund the successful examples (Beinhocker 2005).
The role of policy is indeed one of the “conditionals” to the optimism. The pace of transformation will still be affected by policy: governments can either hinder or help it. Yet, the one word in the above paragraph that I disagree with is “global”. The effort does not have to be global. It just requires a critical mass to drive the transformations required, to the point where they—the technologies, the new economic interests, the collaborations, and the narrative powers of good examples that are driving successful transitions—become self-sustaining.
4. On Energy Demand and the Macroeconomics of Transition
Another important “conditional” in the optimism concerns energy demand—the main focus of Semieniuk et al’s study. Renewable energy remains a small child in the global energy scene. The reason why the IPCC scenarios for 1.5C involved significant reduction in global energy demand over the next couple of decades is because the clean energy growth rates implied even by logistics-type growth of renewables cannot plausibly catch up with growing energy demand in the next couple of decades: squeezing out coal (and then oil) quickly requires rising clean supplies to meet falling overall demand. Is this possible?
I think the honest answer is that we don’t know, and probably therein lies a crucial distinction between “1.5C” vs “2C” type scenarios. This section does not attempt to conduct quantitative analysis, or to replicate the vast detail that has gone into scenario development using more complex models. But it does again seek to present the historical data in a new light; to link the Semieniuk et al analysis with the territorial vs consumption challenge posed by Schröder and Storm; and from this, to pose the key questions.
Both papers engage energy or carbon-GDP relationships and Figure 3 shows two charts that I use to teach my students about this topic. The first shows a classic chart on energy-GDP which purportedly shows a linear relationship using a veritable mass of data on individual country trends over time—which I grey out precisely because it is potentially misleading for at least two reasons.
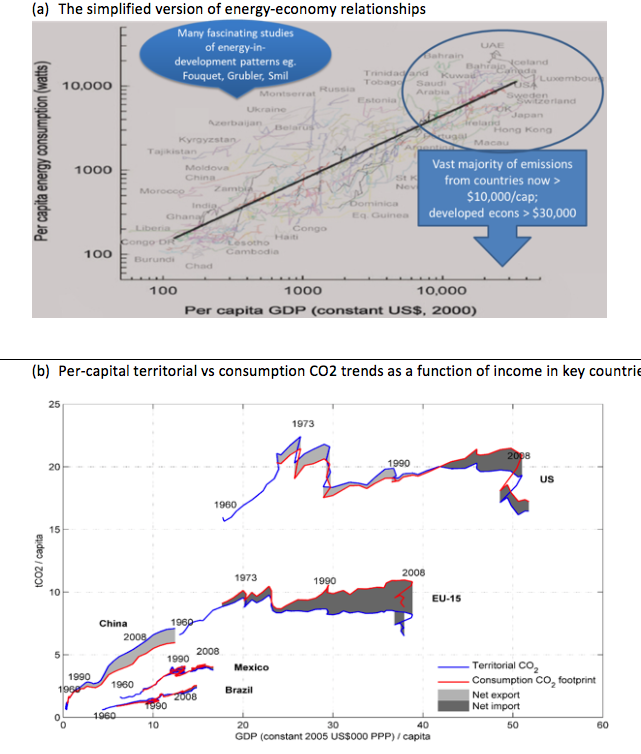
Figure 3: Energy and Carbon Rrends in Relation to GDP Source: (b) As published by the author (Grubb et al 2016) using data www.carboncap.eu
First, it is not really a straight line—assuming that countries with no GDP would consume no energy, the relationship must actually be concave to go through the origin (ie. with declining income-elasticity of energy consumption as countries get richer). Second, if we are worried about global impacts of energy, what matters is wholly dominated by countries with GDP exceeding $10,000/cap±it is the rich and rapidly emerging economies that totally dominate the global total. The rest of the graph is intellectually fascinating but largely irrelevant to climate change—albeit with a caveat flagged below.
As Semieniuk et al note, $10,000/cap happens to be the current global average income. We are in that sense at a threshold, as also implicit in Fig 3(b). What can happen to energy and emissions in those already above this threshold? Aside from the obvious and totally unsustainable levels of US emissions, the dominant impact will hinge on whether the major emerging economies follow the path on up towards such levels; or the EU (and Japanese) examples which emit half as much per person; or is China the exception, with most closer to the Brazilian or Mexican paths? And will those regions currently below the threshold follow historical patterns, or—apparently like Brazil in terms of present energy emissions intensity—find something different? These questions determine our global future.
The fact that the straight line of Fig 3(a) is misleading in terms of territorial emissions is indeed a finding of the studies and data analyzed by both the INET papers. This becomes even clearer when one compares with the second graph, the template for “environmental Kuznets curve” type analysis, which looks to see if energy/emission growth trends reverse at high levels of wealth. The territorial per-capita CO2 emissions of both the US and EU are less today than they were fifty years ago, despite a doubling of GDP. So much for linear energy-GDP relationships.
However as stressed (quite correctly) by Schröder and Storm, part of this is the shift of “embodied” emissions from the rich countries to the poor; the global impact must be considered on a consumption as well as territorial basis. Their analysis finds a “carbon Kuznets curve” type relationship for territorial CO2, but not for consumption-based CO2. Declining rich country territorial emissions is not much use if it is in reality just a transfer of their emissions footprint. It is however a complex field as they acknowledge:
“methodological literature on the CKC does not provide unambiguous and robust evidence of a CKC peaking for carbon dioxide, if only because of well documented but yet unresolved econometric problems concerning the appropriateness of model specification and estimation strategies (e.g. Wagner 2008).
Yet they go on to state:
…the empirical fact that domestic production-based GHG emissions in (for example) the EU have come down, but consumption-based emissions associated with EU standards of livings have actually increased (Peters and Hertwich 2008; Boitier 2012).”
Note the dates. In the spirit of the preceding analysis, we need to look closely at the most recent trends. Most of the sources cited are drawing on datasets that barely reach beyond the financial crisis—which as indicated by Figure 3(b) seems to have marked a major discontinuity with subsequent patterns having economic growth combined with falling consumption emissions. A recent update finds:[7]
.. “we update historical analysis with a novel finding that emission transfers between OECD and non-OECD countries peaked in 2006, and have been declining since. The reversal is principally due to the reduction in the [energy and emissions] intensity of traded goods, rather than the volume of trade.”
As highlighted by Schröder and Storm robust statistical analyses based on limited and evolving data are complex so this may not be a definitive result—but it at minimum suggests that while the growth of consumption-based emissions did indeed reflect growing “outsourcing,” the last decade has seen decline, driven more by reducing energy and emissions intensity of traded goods.
From past to future. Against such background, could energy demand be held constant or reduce—and if so, what might indeed break the main trends of the past? I suggest we need to consider this in terms of key phases and components, drawing on the insight from one of the founders of modern energy demand and efficiency analysis that later stages of economic development comprise largely a shift “from production to pleasure.” Initially, industrial energy demand dominates commercial energy consumption, but it then declines substantially in relative terms in favor of the pursuit of comfort (eg. larger and warmer homes) and other pleasures of personal transport, entertainment and communication.
The energy intensity of “pleasure” is incredibly variable, pliable, and subject to technology. We see a very wide range in the energy intensity of buildings and vehicles, except where combinations of regulation and pricing have forced higher standards. Many consumers pay little attention to their energy consumption precisely because the energy costs of many of these activities are either so low (the worst homes and vehicles aside), or just intangible and unobservable at the point of consumption. This can lead to large structural inefficiencies, compared to what is technically already available. In the terminology of Planetary Economics, energy efficiency is the totemic example of first domain behaviors (satisficing, contractual and principle-agent failures etc.), with large potential for policy-driven improvements. The UK progress in electricity, charted above, is also a story of demand reduction. Moreover, much of our entertainment and communication rests on information technology, and electric cars do not only bring the potential for cleaner fuels, they are intrinsically more efficient still. If there is potential for global demand reduction it may be led by such combined forces, in concert with the IT and AI revolutions. We do not know, but it is unclear how much past global trends are a good guide to the future.
Turning to the “production”—which now accounts for less than half of global energy demandI suggest we need to disentangle two components. One is manufacturing, and particularly, internationally mobile manufacturing—the goods which when traded account for the gap between territorial and consumption based data, in which just half a dozen basic commodities led by steel dominate emissions. There certainly are technical options, ranging from more recycling and the “circular economy” to the use of new materials, as detailed in the IPCC reports. In principle, one could envisage a transition in which major companies re-invent themselves as purveyors of low carbon materials, which replicate the dynamics discussed above to displace carbon-intensive manufacturing.
The greatest case for pessimism lies in systematic failures of policy: the lack of attention to materials efficiency; the fact that without effective carbon pricing, these industries have little incentive to change; and the fact that because of mobile production, policy is politically infeasible or ineffective (or both) without consumption-based pricing or border carbon adjustments. This indeed is full of irony. In the growing trade war between China and the US, it seems the world is unwilling even to think about the entirely legitimate use of consumption-based or border carbon pricing either to encourage cleaner production in China, or to deter the Trump administration from using discriminatory trade measures to re-industrialize drawing partly on older and more carbon-intensive technologies. The failure of governments to even think seriously about incentives for mobile manufactured goods—but instead basically exempt these sectors—is probably the single strongest reason for pessimism. But it is a structural failure of policy, not an intrinsic economic law rooted in past statistics or the lack of future alternatives.
Finally, this discourse has to consider the basic infrastructure, particularly associated with urbanization in developing countries. This is not a trade issue—but it is an international one. As the chief executive of the world’s biggest cement company observed, “we know how to make very low carbon cement – by why would we? There is no incentive.” This, centrally, combines the need for carbon pricing in energy intensive industries with effective support and standards for those regions still struggling to establish basic infrastructure. If forward-looking, good infrastructure could also aid from the outside the kind of very low carbon, green space and electric-transport-based cities of the kind now being pursued in some Chinese pilots like Langing.
Thus again: there are examples. Optimism would be rooted in the potential for new industrial processes and niche examples to be so attractive as to rapidly grow and spread. Pessimism is rooted in the evidence to date that policy—renewable incentives aside—is more timid and more resistant to change than technology itself. But in neither case, I would argue, do the statistics of past aggregate trends really indicate the constraints on what is possible.
5. Conclusion: Four Principles for “Historical Futures” Analysis
One fascinating contrast between the two papers reviewed here concerns their macroeconomic perspectives. Again with admirable clarity, Semieniuk et al note:
There is doctrinal dispute among economists about whether output in the medium run is set by forces of supply (typically assumed to involve full employment of labor and determination of investment in new capital by available saving) or whether it responds to effective demand (full employment not assumed and saving follows investment). Our model incorporates the latter assumptions. Outlays on mitigation add to demand, and so generate more output and employment.
This does indeed lead them to view the dominant constraints as the political challenge of shifting and enhancing investment, which however has potential to boost growth.
In contrast, Schröder and Storm, whilst acknowledging all the optimism of the “green growth” literature, structure their Kaya identity as implying that going beyond historical rates of change means curtailing growth. They note that “Some scholars argue that radical de-carbonization will not be possible while increasing the size of the economy.” To use a non-academic phrase, this is bunk: the most pessimistic studies of classical economics find that in low carbon scenarios we would reach similar levels of global wealth just a year or two later, they just tend to avoid mentioning the fact. More provocatively, we could interpret Semieniuk et al as implying that radical decarbonization will in contrast not be possible without increasing the size of the economy, fueled and funded by a new industrial revolution in energy and its efficient use.
Rather than entering further into this debate, I return to the basic approach of the papers. Whilst to some degree reflecting standard econometric approaches, in effect, we might term that both papers adopt an approach of “historical futures” analysis. As said, trying to read the runes of past statistics is an advance on the use of models built on the quicksands of inappropriate theory and largely random (and now mostly invalidated) assumptions about future costs.
But we need to consider more deeply what we are looking for and how we might identify it. Before declaring that history has set limits on what is possible, we need to be extremely careful. The future has already started, though its beginnings may be modest. And to read the runes correctly, I suggest four principles for such ‘historical futures’ analysis of energy-climate challenges.
- The future may be found not in aggregate statistics of past emissions, but in the technology- and or country-specific examples that are successful and growing
- “Historical future” analysis should consider transitions in terms of logistic substitutions, not extrapolated % rates-of-change of the macro-energy-economic variables (of energy or carbon intensity / productivity).
- More data is not always better. If we are concerned about the prospects for low carbon energy systems, the future started in about 2000 in terms of indicators observable in most energy statistics.
- Both aggregate and trade dimensions since then have however been heavily influenced by the almost unprecedented pace, carbon intensity and scale of the Chinese economy: analysis thus needs to consider independently the Chinese impact before generalizing global conclusions.
My central argument though is simpler: following Romer, that we are not slaves of the past, in the sense that econometric and historical studies suggest. We have already gathered many of the materials we need, and with a right guidebook, we can still be the builders of our future.
I am grateful to Dimitri Zhengelis for pointing out this link as a lovely rendition of the apparently esoteric – but absolutely fundamental – difference between the economics of exogenous and endogenous technical change. Romer also notes that productivity growth rates have been faster for countries developing later, relevant to both supply and demand-side forecasts.
For the most recent data at the time of writing this article, see https://www.lazard.com/media/4…
Global electricity consumption is about 22,000TWh/yr. Solar PV by 2016 was supplying an estimated 400TWh, or 0.2% of global consumption (much lower than the relative installed capacity since PV output is only 10-20% of installed capacity). To be precise, 0.18% in 2017, compounded at 30%/yr for 24 years reaches 98%.
[4] For a detailed analysis see Grubb and Newbery (2018), report for MIT (2018a) subsequently developed and published in The Energy Journal (2018b).
In fact this is rather conservative, yielding a t0 = 2015 renewables penetration of about 15% (MAX / (1+a) = 50% / 3.5 = 14.3%, plus tiny additional element from ‘system’ technologies in this representation), significantly below that actually achieved for renewables overall, but close to that of wind & solar combined, which supplied 14.2% of UK electricity in 2015 but already 18.3% by 2017 (DUKES, Table 5.1)
[6] Source: Veloz. The California Air Resources Board has a target of 5m electric vehicle sales by 2030, which with annual sales growth at 30%/yr would indeed be the annual sales rate in 2030.
[7] Unfortunately this paper (by Wood et al) is in review so I am not sure will be citable in published version